This chronicle follows the causal arc from biodiversity loss through to increasing pandemics, putting at risk the most vulnerable sectors of society, including the very indigenous people who are some of the best custodians of biodiversity. We review evidence of the links between the destruction of the environment and disease emergence and expand the discussion to cover the potential for epidemics to arise in the Amazon.
Forlorn Warning
Researchers looking at the links between the health of ecosystems, animals and humans have foretold of a pandemic for years (Fan et al. 2019). The who, what, where and when of the current, surreal drama in which we have all unwittingly become pivotal characters in central casting were a mystery. But the stage was set on the ‘how’ decades ago.
The alarm raised by scientists mostly fell on deaf ears, until crisis hit.
The story begins with human encroachment into natural landscapes. Across the world, industrial activities such as agriculture, ranching, hydroelectric dam construction, urbanization, logging, oil extraction and mining are replacing earth’s original biomes with ones in which humans dominate. 76% of the earth’s land has already been altered, leaving only 23% to wilderness (Watson et al. 2018). This is the setting…
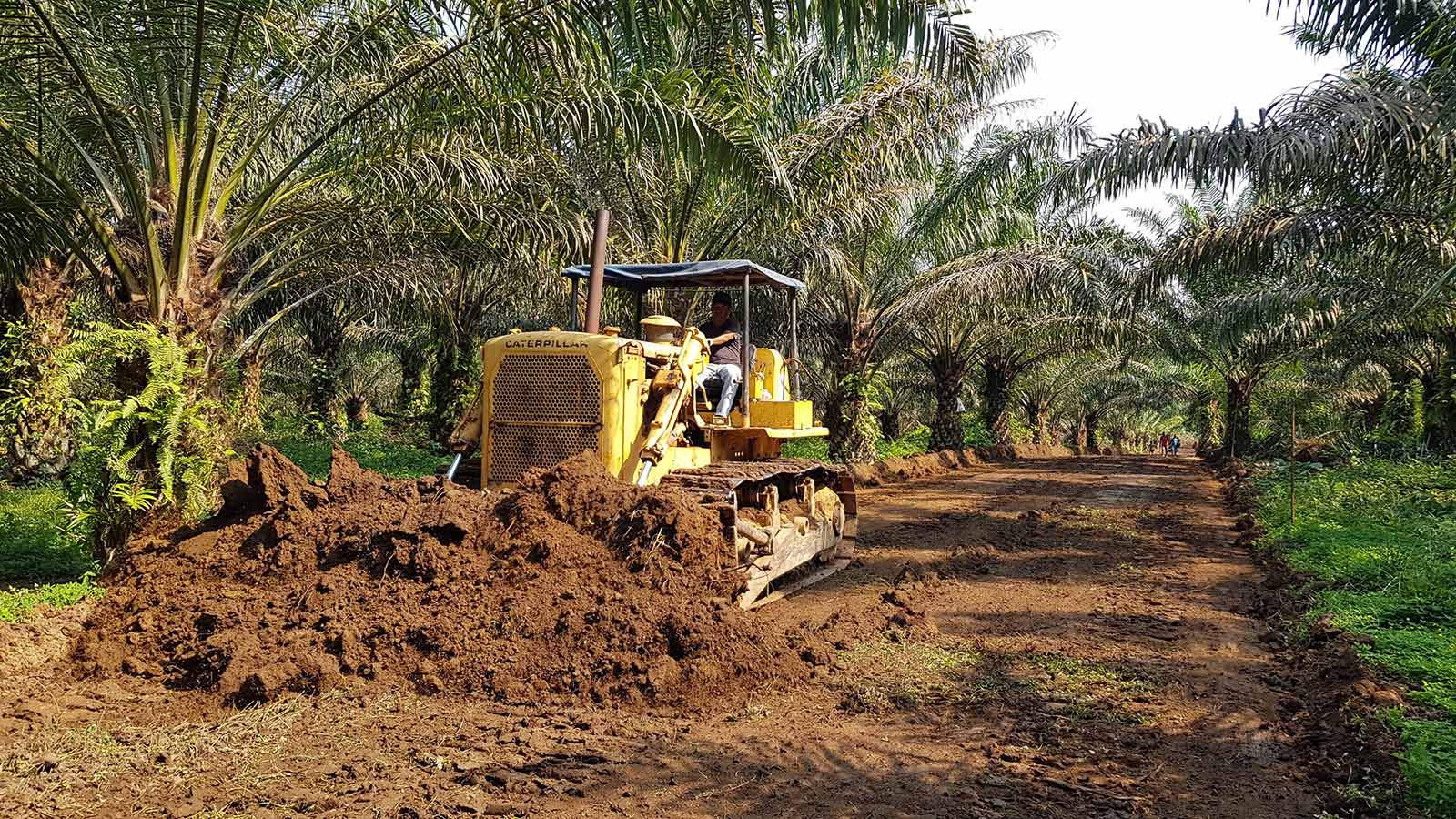
Palm oil production is fast becoming one of the main drivers of deforestation in parts of the Amazon, causing a myriad of health problems
…now, the scenario. Land degradation provokes biodiversity loss, which in turn facilitates the propagation of diseases. Here is how it plays out. Diverse ecosystems harbor high biodiversity with significant genetic diversity. The genetic diversity of potential victims hampers the spread of disease by consistently posing unique challenges to would-be-killers (van Houte et al., 2016). If the genetic diversity of the potential target animal is high it will be harder to find a foolproof way to circumvent the immune system designed to thwart it and sneak past the cellular wall. On the other hand, if the gene pool is low, as it is when animal biodiversity is lost, pathogens quickly find solutions to dupe hosts’ immune systems and then spread rapidly throughout the relatively uniform population.
Enter HUMANS. Meanwhile, landscapes where humans dominate lead to a greater proportion of species that can tolerate the presence of humans, such as rodents and bats, as the more reclusive types slink deeper and deeper into the remaining wilderness. Exit more BIODIVERSITY. Furthermore, some of the characteristics that make these species adaptable to human environments also make them excellent hosts for infectious diseases: high mobility, longevity, and social living in colonies (Mühldorfer, 2013). As demonstrated by indigenous people across the globe, we do have the capacity to occupy spaces without dominating and degrading them: 80% of the world’s biodiversity resides inside traditional indigenous territories (Sobrevilia, 2008). But when human activities run amok and push really comes to shove, it’s Mother Nature who has the last words: Back off!
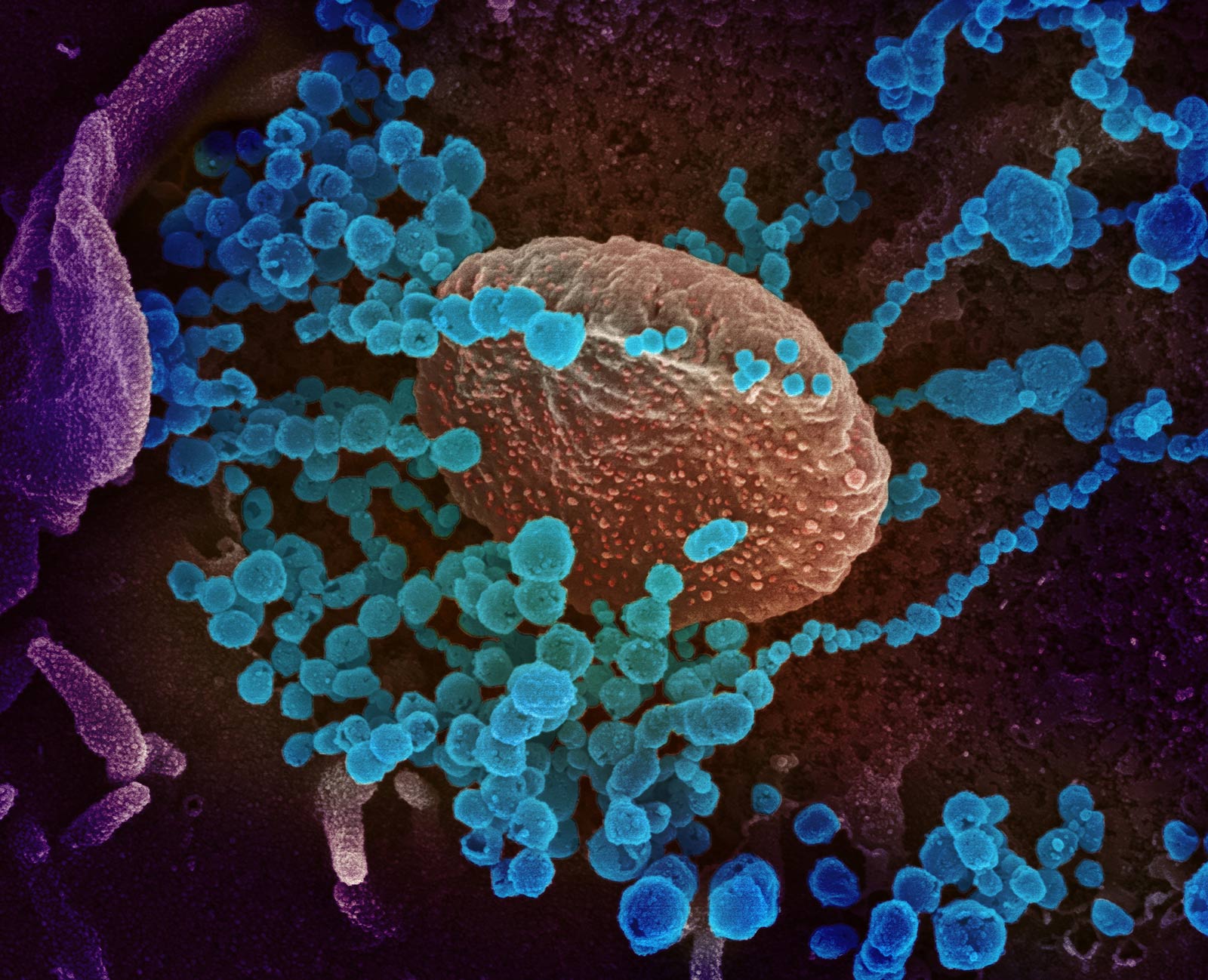
This scanning electron microscope image shows SARS-CoV-2 (round blue objects) emerging from the surface of cells cultured in the lab. SARS-CoV-2, also known as 2019-nCoV, is the virus that causes COVID-19. The virus shown was isolated from a patient in the U.S. Credit: NIAID-RML
‘Whodunit’
Here’s where the plot thickens. As humans inhabit these shifting landscapes containing less and less biodiversity they come into contact with unforeseen pathogen hosts. The most common suspects are either domesticated animals or those sociable, adaptable, human tolerant characters, the rodents and bats. Original hosts develop immunity to pathogens over eons, carrying them unknowingly. Spillover events – when a pathogen adapts to use a new host – sometimes lead to permanent host shifts and are common in evolutionary history (Longdon et al. 2014). This is the making of an epidemic, because the new host has no immunity to the “novel” virus.
Coronaviruses, which are a large class of viruses normally found in rodents, bats and birds, cannot directly affect humans, and must pass through an intermediary host first. In a foreshadowing of what is coming to pass with COVID-19, SARS took center stage in 2002 when this coronavirus moved from bat to civet, a small nocturnal mammal, before becoming virulent for humans. Avian and swine flu often originate in domesticated animals. The H1N1 epidemic of 2009 has been traced to central Mexico and is believed to be the result of long-distance live pig travel allowing several strains of virus to recombine, generating a bug that could inflict harm on humans (Mena et al. 2016).
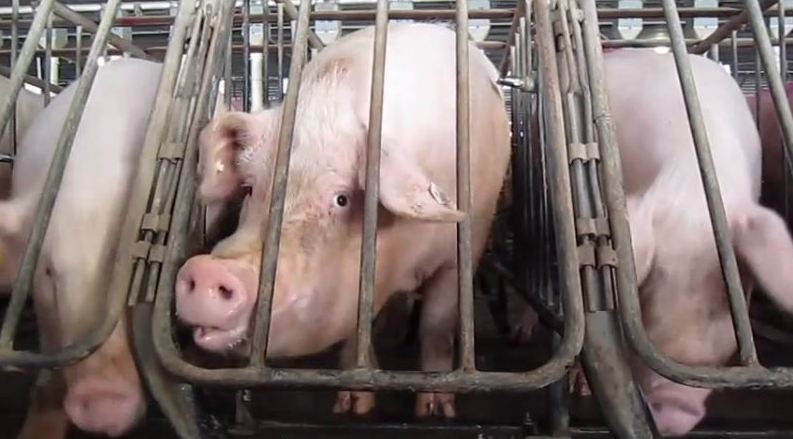
Smithfield Foods gestation crates, Smithfield Foods/Murphy Brown pig breeding facility, Waverly, Virginia, United States. Photo Courtesy of Humane Society of the United States
The “Spanish” flu of 1918 infected 500 million and killed between 50 and 100 million people (Sutton, 2018). The origins of this H1N1 virus are murky, but there was certainly a crossover from birds to humans, likely involving pigs as intermediary hosts (van Wijhe et al. 2018). Interestingly, it is believed that this virus was able to attain pandemic proportions despite the simplified demographics of the time due to troop movements throughout Europe and back to North America during WWI (van Wijhe et al. 2018). Few places escaped the pandemic, which reached its tendrils into the remotest regions of the world, including the Amazon (Chowell et al. 2011).
Scientists are furiously investigating how the SARS-CoV-2 virus jumped the species barrier to arrive in humans causing COVID-19. Inquiries have revealed that the original host is most certainly a bat. Molecular studies are pointing to the intermediary host being either pangolin – an endangered mammal traded illegally in wet markets of Asia – snakes or turtles, which are both legally sold at market (Liu, et al. 2020). This fact points to the primacy of clamping down on the illegal trade of wildlife and tightening up laws where they are lax, especially with respect to the sale of live animals at ‘wet’ markets.
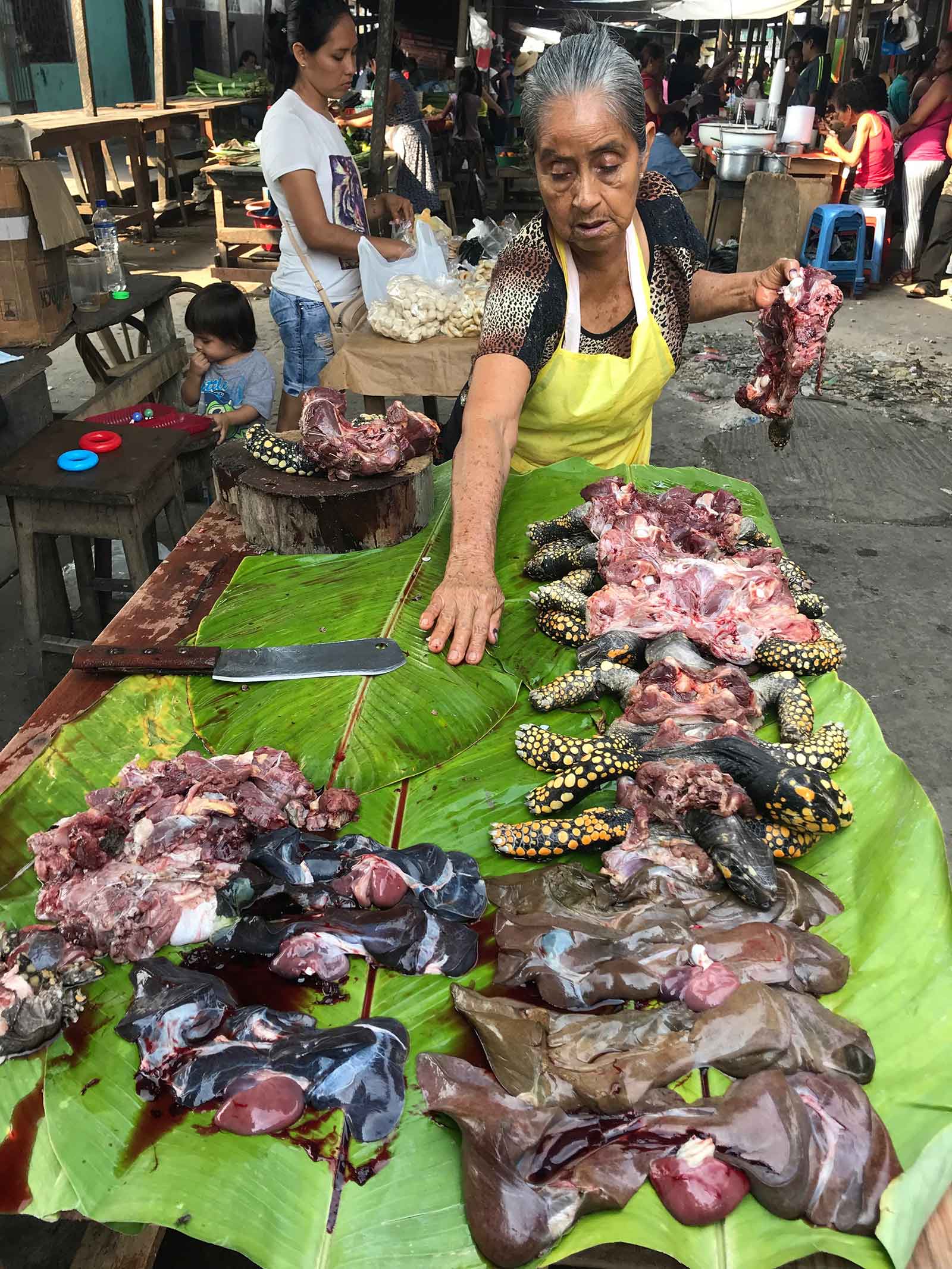
Albeit on a lesser scale than in Asia, wet markets abound in urban centers around the Amazon (pictured above, the Belén market in the city of Iquitos, Peruvian Amazon). There, you can purchase a vast array of bush meat and exotic animals for pets. Live markets are ideal venues for humans to come into contact with potential pathogen hosts
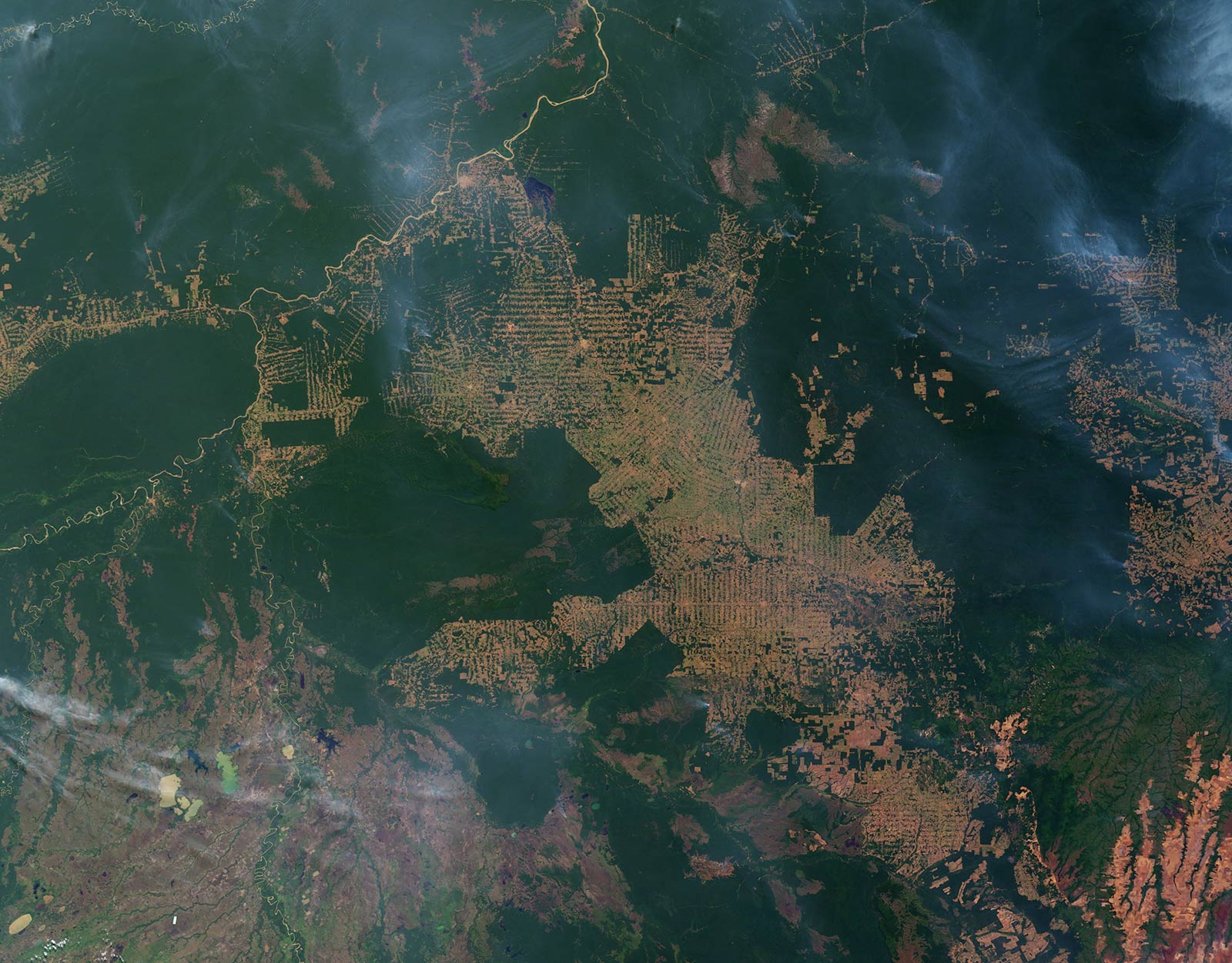
Fires and deforestation on the Amazon frontier, Rondonia, Brazil. Aerial view on August 12 2007 as captured by NASA Earth Observatory
Burning the Amazon: Igniting a future pandemic?
The 2019 dry season in the Amazon had the world on the edge of its seat as unfathomable acreage of pristine rainforest and previously cleared forest burned at the hands of land owners hungry for more land on which to grow cash crops, such as soy and cattle. All of this charred land is on the ancestral territories of the Amazon’s original people; however, it is unclear how much of the burned area was on land currently under indigenous jurisdiction. Contrary to elsewhere in the world, the main driver of land use change in Brazil is rising affluence, not poverty or population growth (Nava et al., 2017). In this sense, avarice is the principal motor of land clearing, while fire is the vehicle, setting in motion biodiversity loss and potentially providing the spark for a spillover event.
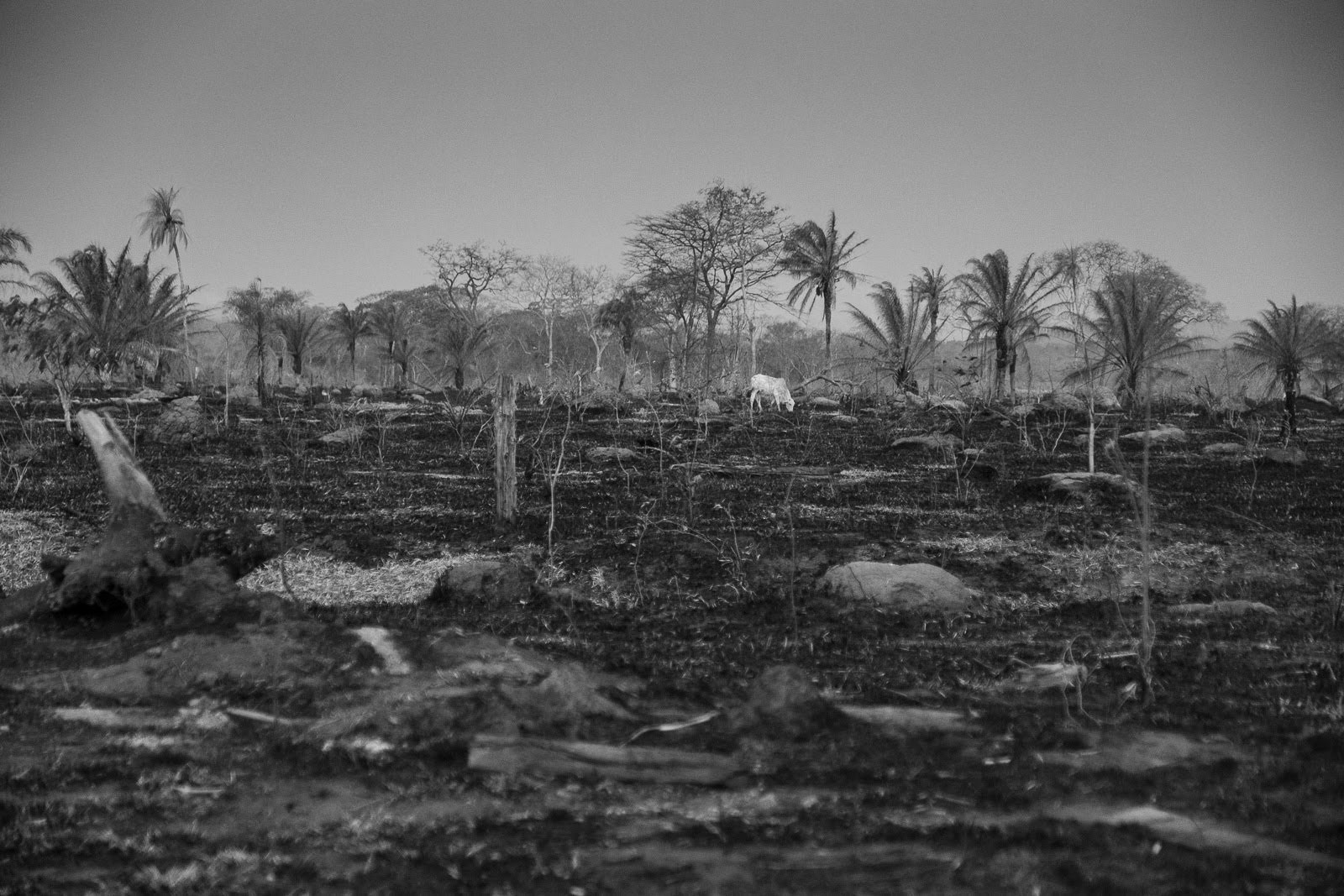
Aftermath of fires in the Bolivian Amazon, August 2019
The vast majority of emerging zoonotic diseases in South America are vector-borne diseases, such as malaria, dengue, chikungunya, and zika, which require a vector, like a mosquito, to infect humans. COVID-19 is an ‘air-borne’ infectious disease. Vector-borne diseases are dependent on the ecological niche of the vector (temperature range, humidity, etc.) making it much less likely that they would reach pandemic scale; whereas airborne diseases travel easily from one human to the next in a wider range of habitats.
Greater Spear-nosed Bat (Phyllostomus hastatus), La Selva Biological Station, Costa Rica. Photo Courtesy of Karin Schneeberger
Research along the deforestation arc of Brazil, the world’s largest deforestation frontier, has demonstrated that bat diversity is significantly lower than in the rest of the Amazon. Two species, the greater spear-nosed bat, Phyllostomus hastatus, and the gnome fruit-eating bat, Dermanura gnoma, were found to have antibodies signaling that they are hantavirus carriers (Sabino-Santos Jr, et al. 2019).
Fires further aggravate the problem because as fields and forests are lit, rodents flee flames taking shelter in dwellings, where humans come into direct contact with them (Laerte Pinto et al. 2014). Bats are also disturbed by fires. Prior to the deadly Nipah outbreak in Asia in 1997-1998, smoke from severe forest fires drove fruit eating bats from the forest into orchards, where a novel virus was forged upon recombination with pig viruses (Bonilla-Aldana et al. 2019). Climate change also contributes to diminishing biodiversity: projections show that, as it heats up and dries out, large swaths of the Amazon could shift to savannah-type landscapes, which house considerably less wildlife than tropical rainforests.
Furthermore, very preliminary data coming out of Italy is leading some scientists to believe that air pollution acts as a carrier for COVID-19. Viruses hitchhike on the particles in the air, facilitating its spread. Previous research has shown that dust storms (Chen et al., 2010) and haze (Ye et al., 2016) act as propagation mechanisms for other respiratory illnesses. If this is the case, COVID-19 or a potential hantavirus outbreak could be widely broadcast by the next Amazon fire season. The lower fauna richness in general, proliferation of human tolerant animals and proximity with settlers following deforestation through burning, would constitute significant risk factors for possible disease emergence in the Amazon. And indigenous people, who are in the firing line between settlers and the falling forest, could be the first to fall victim to an Amazonian virus making its debut.
Several recent deaths in Ecuadorian indigenous Shuar and Siekopai communities are suspected to be COVID-19 related, while in Brazil, there have been at least three COVID-19 deaths and 27 confirmed cases among indigenous people to date.
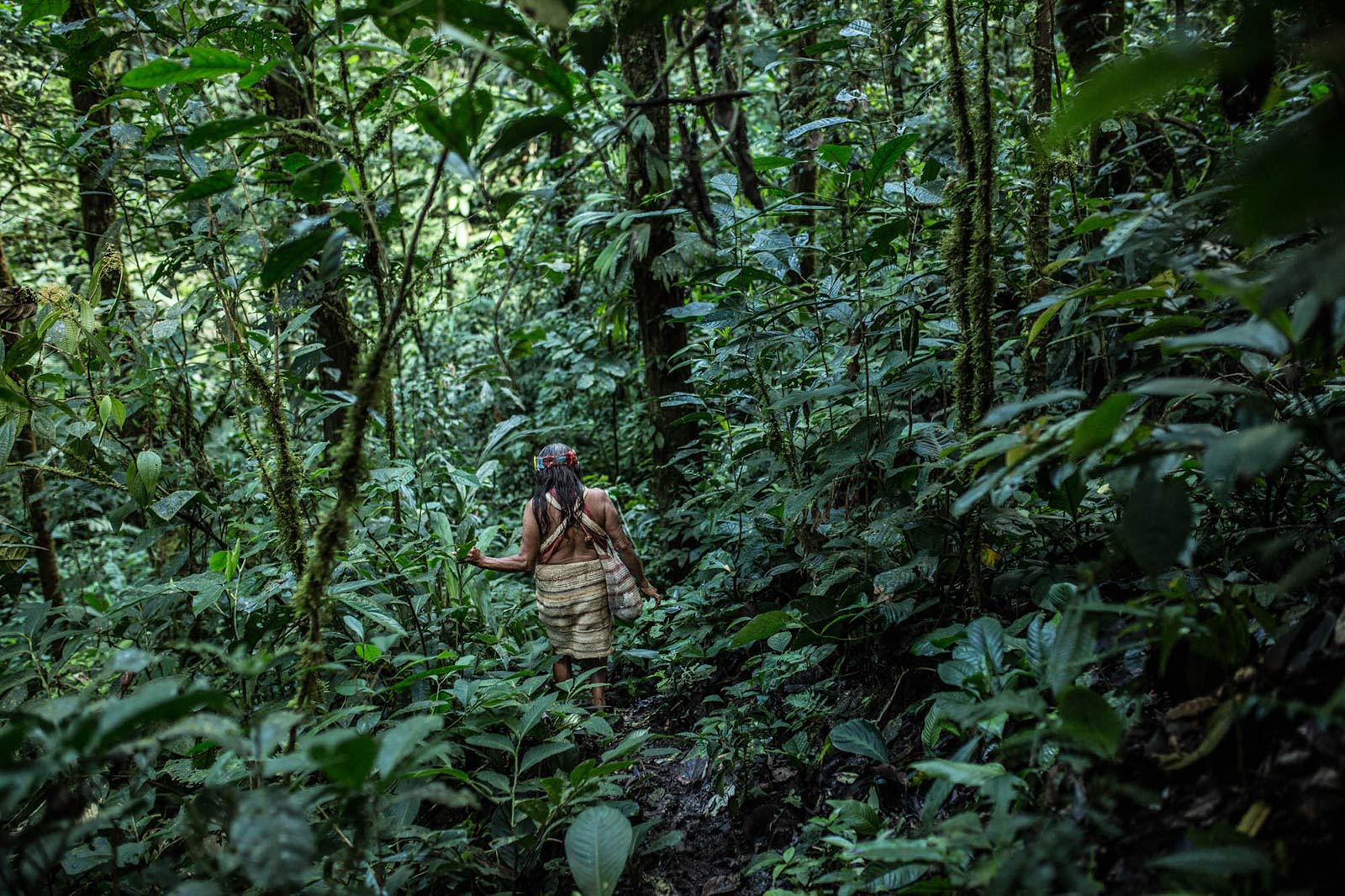
Waorani elder in the forest, Ecuadorian Amazon
The specter of new pandemics adds one more motive in our arsenal of reasons to conserve intact forests. One way of doing this is to slow down resource extraction and agricultural expansion in a post COVID-19 era. Already, indigenous peoples in the Amazon have been demanding an immediate moratorium on any activity that includes the entrance of foreigners into indigenous territories, mining activities, logging, oil exploration and extraction, industrial agriculture, religious proselytization, or increased militarization, especially in trans-border territories under pressure from armed actors and organized crime.
Unfortunately, in some places companies and desperados are taking advantage of the dire situation to push ahead with controversial activities including mining. Meanwhile, in the Brazilian Amazon, indigenous people have witnessed an increase in illegal activities and reduced law enforcement. This lapse in vigilance could exacerbate the 2020 fire season, and as we have seen, fires in the Amazon pose a particularly volatile situation in which diseases could emerge and propagate rampantly among some of the world’s most vulnerable people. However, the COVID-19 crisis has, in other instances, temporarily paused many of these industrial activities, fugitives on the lam, and, in some places, encouraging signs of cleaner air and animals repossessing key habitats are emerging. These beacons show us that another world is possible. Indigenous land management practices are another lodestar: 40% of the world’s protected and natural spaces overlap with indigenous lands (Garnett et al. 2018), highlighting that their management strategies simultaneously allowed for their own survival and the survival or abundant biodiversity.
We still have time to contain the impact of COVID-19 on indigenous communities and avoid a catastrophic loss of life. Please donate to the Amazon Emergency Action Fund to help indigenous communities get information, supplies and medical services to protect themselves during this crisis.
Reference.
- Bonilla-Aldana, D. K., J. A. Suárez, C. Franco-Paredes, S. Vilcarromero, S. Mattar, J. E. Gómez-Marín, W. E. Villamil-Gómez, J. Ruíz-Sáenz, J. A. Cardona-Ospina, S. E. Idarraga-Bedoya, J. J. García-Bustos, E. V. Jimenez-Posada and A. J. Rodríguez-Morales (2019). “Brazil burning! What is the potential impact of the Amazon wildfires on vector-borne and zoonotic emerging diseases? – A statement from an international experts meeting.” Travel Medicine and Infectious Disease 31: 101474.
- Chen, P.-S., F. T. Tsai, C. K. Lin, C.-Y. Yang, C.-C. Chan, C.-Y. Young and C.-H. Lee (2010). “Ambient Influenza and Avian Influenza Virus during Dust Storm Days and Background Days.” Environmental Health Perspectives 118(9): 1211-1216.
- Chowell, G., C. Viboud, L. Simonsen, M. A. Miller, J. Hurtado, G. Soto, R. Vargas, M. A. Guzman, M. Ulloa and C. V. Munayco (2011). “The 1918-1920 influenza pandemic in Peru.” Vaccine 29 Suppl 2(Suppl 2): B21-B26.
- Garnett, S. T., N. D. Burgess, J. E. Fa, Á. Fernández-Llamazares, Z. Molnár, C. J. Robinson, J. E. M. Watson, K. K. Zander, B. Austin, E. S. Brondizio, N. F. Collier, T. Duncan, E. Ellis, H. Geyle, M. V. Jackson, H. Jonas, P. Malmer, B. McGowan, A. Sivongxay and I. Leiper (2018). “A spatial overview of the global importance of Indigenous lands for conservation.” Nature Sustainability 1(7): 369-374.
- Fan, Y., K. Zhao, Z.-L. Shi and P. Zhou (2019). “Bat Coronaviruses in China.” Viruses 11(3).
- Mühldorfer, K. (2013). “Bats and Bacterial Pathogens: A Review.” Zoonoses and Public Health 60(1): 93-103.
- Laerte Pinto, V. J., H. Amani Moura, F. Dalcy de Oliveira Albuquerque and S. Vitorino Modesto dos (2014). “Twenty years of hantavirus pulmonary syndrome in Brazil: a review of epidemiological and clinical aspects.” The Journal of Infection in Developing Countries 8(02).
- Liu, Z., X. Xiao, X. Wei, J. Li, J. Yang, H. Tan, J. Zhu, Q. Zhang, J. Wu and L. Liu (2020). “Composition and divergence of coronavirus spike proteins and host ACE2 receptors predict potential intermediate hosts of SARS-CoV-2.” Journal of Medical Virology n/a(n/a).
- Longdon, B., M. A. Brockhurst, C. A. Russell, J. J. Welch and F. M. Jiggins (2014). “The evolution and genetics of virus host shifts.” PLoS pathogens 10(11): e1004395-e1004395.
- Nava, A., J. S. Shimabukuro, A. A. Chmura and S. L. B. Luz (2017). “The Impact of Global Environmental Changes on Infectious Disease Emergence with a Focus on Risks for Brazil.” ILAR Journal 58(3): 393-400.
- Mena, I., M. I. Nelson, F. Quezada-Monroy, J. Dutta, R. Cortes-Fernández, J. H. Lara-Puente, F. Castro-Peralta, L. F. Cunha, N. S. Trovão, B. Lozano-Dubernard, A. Rambaut, H. van Bakel and A. García-Sastre (2016). “Origins of the 2009 H1N1 influenza pandemic in swine in Mexico.” eLife 5: e16777.
- Sabino-Santos Jr, G., F. F. Ferreira, D. J. F. da Silva, D. M. Machado, S. G. da Silva, C. S. São Bernardo, M. dos Santos Filho, T. Levi, L. T. M. Figueiredo, C. A. Peres, R. V. de Morais Bronzoni and G. R. Canale (2019). “Hantavirus antibodies among phyllostomid bats from the arc of deforestation in Southern Amazonia, Brazil.” Transboundary and Emerging Diseases: 1– 7.
- Schneider, M. C., P. C. Romijn, W. Uieda, H. Tamayo, D. F. d. Silva, A. Belotto, J. B. d. Silva and L. F. Leanes (2009). “Rabies transmitted by vampire bats to humans: an emerging zoonotic disease in Latin America?” Revista Panamericana de Salud Pública 25(3): 260-269.
- Sobrevila, C. (2008). The Role of Indigenous Peoples in Biodiversity Conservation: The Natural but Often Forgotten Partners. Washington, D.C. , The International Bank for Reconstruction and Development / THE WORLD BANK.
- Sutton, T. C. (2018). “The Pandemic Threat of Emerging H5 and H7 Avian Influenza Viruses.” Viruses 10(9): 461.
- van Houte, S., A. K. E. Ekroth, J. M. Broniewski, H. Chabas, B. Ashby, J. Bondy-Denomy, S. Gandon, M. Boots, S. Paterson, A. Buckling and E. R. Westra (2016). “The diversity-generating benefits of a prokaryotic adaptive immune system.” Nature 532(7599): 385-388.
- van Wijhe, M., M. M. Ingholt, V. Andreasen and L. Simonsen (2018). “Loose Ends in the Epidemiology of the 1918 Pandemic: Explaining the Extreme Mortality Risk in Young Adults.” American Journal of Epidemiology 187(12): 2503-2510.
- Watson, J. E. M., O. Venter, J. Lee, K. R. Jones, J. G. Robinson, H. P. Possingham and J. R. Allan (2018). “Protect the last of the wild: Global conservation policy must stop the disappearance of Earth’s few intact.” Nature 563: 27-30.
- Ye, Q., J.-f. Fu, J.-h. Mao and S.-q. Shang (2016). “Haze is a risk factor contributing to the rapid spread of respiratory syncytial virus in children.” Environmental Science and Pollution Research 23(20): 20178-20185.